NERSC Powers Insight Into Chemical Actuation of Self-Folding Origami Machines
New approach yields more efficient method for converting chemical energy into mechanical work
July 31, 2023
By Keri Troutman
Contact: cscomms@lbl.gov
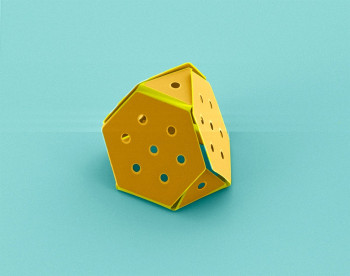
This scanning electron microscope image shows an origami tetrahedra microstructure that self-folded after it was exposed to hydrogen. Credit: Cornell University
Science Breakthrough
Inspired by how our body's motor proteins facilitate tasks like muscle contraction and cellular migration, scientists have been looking for efficient ways to convert chemical energy (the energy stored in substances) into useful mechanical work. By understanding and harnessing the mechanisms that nature uses, we can develop new technologies and advancements with implications in areas like energy production, transportation, robotics, and more. However, finding efficient methods of doing this has been challenging. Many existing methods need to be faster and require extreme conditions to work properly. So scientists are looking for better ways to achieve this conversion efficiently, to more closely mimic how nature does it inside our cells.
Toward this end, a team of researchers from Cornell University and the University of Wisconsin-Madison discovered a technique that harnesses chemical reactions to make microscale origami machines; similar to the ancient Japanese art of folding paper to create intricate and complex shapes, these machines can fold in on themselves quickly to take on a multitude of forms. Three-dimensional microfabrication based on origami-inspired materials is a promising technique for building materials, structures, devices, and systems with unconventional electronic, mechanical, and optical properties. This research could lead to autonomous material systems governed by the material's response to its own chemical composition, eventually transitioning into systems that go beyond the limitations imposed by natural microactuation systems. (Microactuators are small-scale active devices that can generate mechanical motion of solids or fluids.)
Science Background
When scientists design devices that can convert chemical reactions into movement – known as chemomechanical transduction – they usually use indirect methods by harnessing physical effects caused by the reaction. These effects work only in liquids and require precise temperatures. But for this study, the researchers used NERSC’s Perlmutter and Cori supercomputers and electronic structure calculations to figure out how to control the speed of reactions happening on surfaces. Their bioinspired chemical strategy involved using ultrathin platinum sheets to generate forces that directly drive microactuation, causing small movements in the devices and eliminating the need for temperature control, allowing them to operate in normal room-temperature conditions.
Using this approach, they found a crucial moment in the process: when oxygen atoms strip hydrogen atoms, it causes the atomically thin material to deform and bend like a hinge. This can integrate photochemically controlled reactions and be used to drive the reconfiguration of microhinges and complex origami- and kirigami-based microstructures, according to Manos Mavrikakis, a professor of chemical and biological engineering at UW-Madison who co-authored the paper and managed the computations run at NERSC.
Science/Computing Breakdown:
Using the Vienna Ab initio Simulation Package (VASP), a computer program designed for atomic-scale materials modeling that helps with electronic structure calculations and quantum-mechanical-based molecular dynamics, the researchers looked closely at the identity of the atoms in the molecules to determine how to vary the bending on the surface. By following the cycle of the actuation that is catalyzed by platinum, they found that between bent and straight states, the chemical energy from the molecules is transformed in the gas phase to mechanical energy. Depending on the molecules and the chemical reactions that can be used for transition between these two states, the scientists found that they could modulate the response time to 600 milliseconds per cycle. Until now, only biological systems have been able to convert chemical energy to mechanical work at these timescales, Mavrikakis noted.
This was very computationally intensive work that couldn’t have been achieved without the additional GPUs on NERSC’s Perlmutter system, Mavrikakis noted.
Looking ahead, the research team is working to transition into systems that go beyond the limitations imposed by the natural microactuation systems, he added, emphasizing that this was just the first example and there could be other options with different types of reactions and different materials to catalyze chemical reactions.
Research Lead
Nicholas Abbott, Cornell University
Co-authors
Nanqi Bao, Qingkun Liu, Michael F. Reynolds, Marc Figueras, Evangelos Smith, Wei Wang, Michael C. Cao, David A. Muller, Manos Mavrikakis, Itai Cohen, Paul L. McEuen
Publication:
Gas-phase microactuation using kinetically controlled surface states of ultrathin catalytic sheets
The Proceedings of the National Academy of Sciences, May 1, 2023, 120 (19) e2221740120, https://doi.org/10.1073/pnas.2221740120
Related links
Self-folding origami machines powered by chemical reaction
Funding
This work was supported by the Cornell Center for Materials Research (DMR-1719875), the Army Research Office (ARO W911NF-18-1-0032), the NSF (EFMA-1935252) the Air Force Office of Scientific Research (MURI: FA9550-16-1-0031), and the Kavli Institute at Cornell for Nanoscale Science. The theoretical work was supported by Department of Energy Basic Energy Sciences, Division of Chemical Sciences, grant DE-FG02-05ER15731.
User Facilities
NERSC
About NERSC and Berkeley Lab
The National Energy Research Scientific Computing Center (NERSC) is a U.S. Department of Energy Office of Science User Facility that serves as the primary high performance computing center for scientific research sponsored by the Office of Science. Located at Lawrence Berkeley National Laboratory, NERSC serves almost 10,000 scientists at national laboratories and universities researching a wide range of problems in climate, fusion energy, materials science, physics, chemistry, computational biology, and other disciplines. Berkeley Lab is a DOE national laboratory located in Berkeley, California. It conducts unclassified scientific research and is managed by the University of California for the U.S. Department of Energy. »Learn more about computing sciences at Berkeley Lab.