Berkeley Lab Helps Fuel Advances for Renewable Energy Sources
Computations Run at NERSC Shed Light on an Artificial-Photosynthesis Mystery
April 7, 2022
By Patrick Riley
Contact: cscomms@lbl.gov
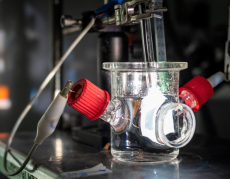
A model solar fuels device called a photoelectrochemical cell developed at Berkeley Lab. (Credit: Thor Swift/Berkeley Lab)
Simulations run at the National Energy Research Scientific Computing Center (NERSC) at Lawrence Berkeley National Laboratory (Berkeley Lab) could enhance the development of a new artificial photosynthesis device component – a promising step forward in validating the viability of renewable fuels.
In findings published in Nature Energy, a team of researchers from Berkeley Lab revealed how the device degrades with use, and demonstrated ways to mitigate the degradation.
For this study, the team designed a model solar fuels device, known as a photoelectrochemical (PEC) cell, made of copper oxide or cuprous oxide (Cu2O). Although cuprous oxide is among the best potential materials for artificial photosynthesis, it breaks down within just a few minutes of exposure to light. To achieve sustainable production of solar fuels and chemicals, the researchers needed to understand the chemical changes at play.
This first involved running a series of simulations on NERSC’s Cori supercomputer, which helped validate the experimental work and vice versa, said Fan Zheng, a co-author on the Nature Energy paper and a former postdoctoral researcher at Berkeley Lab who did most of the computational work. As a result, the research team was able to unravel how cuprous oxide photoelectrodes change under reaction conditions and thus provide a protection scheme to mitigate degradation. The new PEC device contains a heterojunction consisting of iron oxide, tungsten oxide, and gold interfacing with cuprous oxide, where photogenerated charge carriers flow within the heterojunction system, similar to what happens in natural photosynthesis.
The study also provided new insight into how electrons and charge carriers called “holes” contribute to degradation in artificial photosynthesis. Scientists had long assumed that electrons were solely to blame for cuprous oxide’s corrosion. But real-time simulations of the electron and hole dynamics over time showed that holes also play a part.
“Basically we used this scheme to confirm the experimental Z-scheme design and to literally show in a quantum mechanical picture that the design is indeed useful to extract the hole out of cuprous oxide to avoid its degradation,” said Zheng, now a computational materials scientist at ShanghaiTech University.
A Quantum Mechanical Method
The other aim of the computational work was to calculate and show the energy levels of the materials using a quantum mechanical method, he added. That device junction consists of cuprous oxide with gold and tungsten oxide underneath. In this case, researchers ran computer simulations first and then validated them by designing a physical model of an artificial photosynthesis device.
“They measured the energy levels of those materials during the process, and it matched our calculations pretty well,” Zheng said. “We just validated each other in a way.”
The device produced ethylene and hydrogen with unprecedented selectivity and for more than 24 hours, the researchers said, calling it “a thrilling result.”
“We hope that our work encourages people to design strategies that adapt to the intrinsic features of semiconducting materials in artificial photosynthesis devices,” said Guiji Liu, a scientist in the Liquid Sunlight Alliance in Berkeley Lab’s Chemical Sciences Division and first author on the Nature Energy paper.
Because the chemical reactions are happening very fast – in some cases in less than one picosecond – conducting the observational experiments can be tricky, Zheng said. That’s where computational work and simulations can help provide needed backup.
“Indeed, the experimental data we gathered only provided indirect information about the charge transfer route in the reaction. We expected theoretical simulations to help reveal the charge transfer mechanism directly,” Liu added.
1 Million Computer Hours
All in, Zheng estimates that it took about 1 million computer hours over the better part of a year to perform the computational work for the study. Some of the computational work also took place at the Oak Ridge Leadership Computing Facility (OLCF).
Computer simulations increasingly complement experimental observations in materials research and other scientific research areas. That’s partly because even 20 years ago computers were not powerful enough to do so, according to Zheng. Back then researchers were only able to simulate very small unit cells of a crystal with 10 or 20 atoms per unit cell, he said. Now, with the power of supercomputers like Cori, that has changed.
“We can do a simulation with a very large system and very complex physical processes,” Zheng said. The size of the interfaces researchers studied in this case was more than 100 atoms. By using density functional theory and a hybrid functional – mixing the widely used semi-local functional with the more accurate but also more expensive exact exchange functional – the researchers were able to provide a more accurate calculation of the energy levels compared to conventional quantum mechanical methods. They were also able to simulate the excited electron and hole transfer as a function of time.
For Zheng and his colleagues, this study presented an opportunity to test new code they had developed: non-adiabatic molecular dynamics. He talked with Liu, who told him they wanted to validate their experiments through computer simulations.
“That was a good chance to test our code and see if it can solve some real problems,” Zheng said.
Researchers are eyeing cuprous oxide as a promising material for artificial photosynthesis because it is relatively cheap and has suitable light-absorption properties.
“The only disadvantage of cuprous oxide is that it’s not that stable,” Zheng said.
Renewable energy is very important, he added, and he is glad that his computational work helped contribute to potential advances in this field.
NERSC and OLCF are U.S. Department of Energy Office of Science user facilities. The Liquid Sunlight Alliance is a DOE Energy Innovation Hub.
For more details about this study, see this Berkeley Lab news release.
About NERSC and Berkeley Lab
The National Energy Research Scientific Computing Center (NERSC) is a U.S. Department of Energy Office of Science User Facility that serves as the primary high performance computing center for scientific research sponsored by the Office of Science. Located at Lawrence Berkeley National Laboratory, NERSC serves almost 10,000 scientists at national laboratories and universities researching a wide range of problems in climate, fusion energy, materials science, physics, chemistry, computational biology, and other disciplines. Berkeley Lab is a DOE national laboratory located in Berkeley, California. It conducts unclassified scientific research and is managed by the University of California for the U.S. Department of Energy. »Learn more about computing sciences at Berkeley Lab.