Berkeley and Princeton Scientists Watch Stars Explode in 3D
September 16, 2010
Contact: Linda Vu, lvu@lbl.gov, +1 510 486 2402
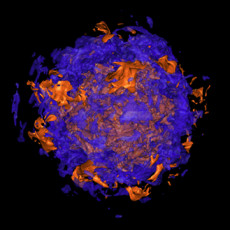
This image shows a slice of a 3D supernova model. The colors show different entropy values. At this snapshot in time, the star is exploding and the shockwave is propagating. Courtesy of Jason Nordhaus and Adam Burrows, Princeton University
For scientists, supernovae are true superstars—massive explosions of huge, dying stars that shine light on the shape and fate of the universe. Recorded observations of supernovae stretch back thousands of years, but only in the past 50 years have researchers been able to attempt to understand what's really happening inside a supernova via computer modeling. These simulations, even crude ones, can lead to new information about the universe's size and eventual fate and help address longstanding problems in astrophysics.
Now, researchers from Princeton University and the Lawrence Berkeley National Laboratory have found a new way to make computer simulations of supernovae exploding in three dimensions. The new simulations are based on the idea that the collapsing star itself is not sphere-like, but distinctly asymmetrical and affected by a host of instabilities in the volatile mix surrounding its core.
Writing in the Sept. 1 issue of the Astrophysical Journal, Jason Nordhaus and Adam Burrows of Princeton University, and the Lawrence Berkeley National Laboratory's Ann Almgren and John Bell report that the new simulations are beginning to match the massive blow-outs astronomers have witnessed when gigantic stars die.
"Visualization is crucial. Otherwise, all you have is merely a jumble of numbers. It allows one to diagnose the dynamics, so that the event is not only visualized, but understood. "—Adam Burrows, Princeton University
The team performed these 3D simulations with approximately 4 million computer processor hours on the National Energy Research Scientific Computing Center's (NERSC) Cray XT4 "Franklin" system. The simulations were run using a sophisticated computer code called CASTRO, the development of which was led by Almgren and Bell of Berkeley Lab's Center for Computational Sciences and Engineering.
"I think this is a big jump in our understanding of how these things can explode," said Burrows, a professor of astrophysical sciences at Princeton. "In principle, if you could go inside the supernovae to their centers, this is what you might see."
In the past, simulated explosions represented in one and two dimensions often stalled, leading scientists to conclude that their understanding of the physics was incorrect or incomplete. Using supercomputers many times more powerful than their predecessors, this team was able to create three-dimensional supernovae simulations, revealing multidimensional instabilities.
"It may well prove to be the case that the fundamental impediment to progress in supernova theory over the last few decades has not been lack of physical detail, but lack of access to codes and computers with which to properly simulate the collapse phenomenon in 3D," the team wrote. "This could explain the agonizingly slow march since the 1960s toward demonstrating a robust mechanism of explosion."
Birth of a supernova
Supernovae are the primary source of heavy elements in the cosmos. Their brightness is so consistently intense that supernovae have been used as "standard candles" or gauges, acting as yardsticks indicating astronomical distances. Most result from the death of single stars much more massive than the sun.
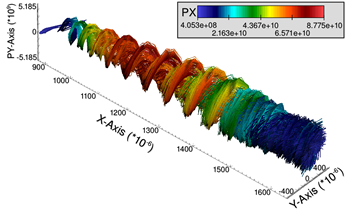
This image shows a 3D time series of the development and expansion of the supernova shock. Time is increasing as you move from left to right. The purple surface is an isocontour of entropy while the blue/green surface is an isocontour of density. Courtesy of Jason Nordhaus and Adam Burrows, Princeton University
As a star ages, it exhausts the hydrogen and helium fuel at its core. With still enough mass and pressure to fuse carbon and produce other heavier elements, it gradually becomes layered like an onion with the bulkiest tiers at its center. Once its core exceeds a certain mass, it begins to implode. In the squeeze, the core heats up and grows even denser.
"Imagine taking something as massive as the sun, then compacting it to something the size of the Earth," Burrows said. "Then imagine that collapsing to something the size of Princeton."
What comes next is even more mysterious.
At some point, the implosion reverses. Astrophysicists call it "the bounce." The core material stiffens up, acting like what Burrows calls a "spherical piston," emitting a shock wave of energy. Neutrinos, which are inert particles, are emitted too. The shock wave and the neutrinos are invisible.
Then, very visibly, there is a massive explosion, and the star's outer layers are ejected into space. This highly perceptible stage is what observers see as the supernova. What's left behind is an ultra-dense object called a neutron star. Sometimes, when an ultramassive star dies, a black hole is created instead.
Scientists have a sense of the steps leading to the explosion, but there is no consensus about the mechanism of the "bounce." Part of the difficulty is that no one can see what is happening on the inside of a star. During this phase, the star looks undisturbed. Then, suddenly, a blast wave erupts on the surface. Scientists don't know what occurs to make the central region of the star instantly unstable. The emission of neutrinos is believed to be related, but no one is sure how or why.
"We don't know what the mechanism of explosion is," Burrows said. "As a theorist who wants to get to root causes, this is a natural problem to explore."
Multiple scientific approaches to solve the problem
This image shows a 3D time series of the development and expansion of the supernova shock. Time is increasing as you move from left to right. The purple surface is an isocontour of entropy while the blue/green surface is an isocontour of density.
The scientific visualization employed by the research team is an interdisciplinary effort combining astrophysics, applied mathematics and computer science. The endeavor produces a representation, through computer-generated images, of three-dimensional phenomena. In general, researchers employ visualization techniques with the aim of making realistic renderings of quantitative information including surfaces, volumes and light sources. Time is often an important component too, allowing researchers create "movies" showing a simulated process in motion.
To do their work, Burrows and his colleagues came up with mathematical values representing the energetic behaviors of stars by using mathematical representations of fluids in motion -- the same partial differential equations solved by geophysicists for climate modeling and weather forecasting. To solve these complex equations and simulate what happens inside a dying star, the team used an advanced computer code called CASTRO that took into account factors that changed over time, including fluid density, temperature, pressure, gravitational acceleration and velocity.
The calculations took months to process on supercomputers at Princeton and Berkeley Lab.
The simulations are not ends unto themselves, Burrows noted. Part of the learning process is viewing the simulations and connecting them to real observations. In this case, the most recent simulations are uncannily similar to the explosive behavior of stars in their death throes witnessed by scientists. In addition, scientists often learn from simulations and see behaviors they had not expected.
"Visualization is crucial," Burrows said. "Otherwise, all you have is merely a jumble of numbers. Visualization via stills and movies conjures the entire phenomenon and brings home what has happened. It also allows one to diagnose the dynamics, so that the event is not only visualized, but understood."
To help them visualize these results, the team relied on Hank Childs of the Berkeley Lab's Visualization Group. Nordhaus noted that Childs played an important role in helping the team create 3D renderings of these supernovae.
"The Franklin supercomputer at NERSC gives us the most bang for the buck," said Nordhaus. "This system not only has enough cores to run our 3D simulations, but our code scales extremely well on this platform. Having the code developers, John Bell and Ann Almgren, nearby to help us scale our simulations on the supercomputer is an added bonus."
This research is supported in part by the Department of Energy's Scientific Discovery through Advanced Computing Computational Astrophysics Consortium led by Stan Woosley of the University of California, Santa Cruz.The National Science Foundation also provided additional support for this work.
This story was adapted from a Princeton University press release written by Kitta MacPherson.
About NERSC and Berkeley Lab
The National Energy Research Scientific Computing Center (NERSC) is a U.S. Department of Energy Office of Science User Facility that serves as the primary high performance computing center for scientific research sponsored by the Office of Science. Located at Lawrence Berkeley National Laboratory, NERSC serves almost 10,000 scientists at national laboratories and universities researching a wide range of problems in climate, fusion energy, materials science, physics, chemistry, computational biology, and other disciplines. Berkeley Lab is a DOE national laboratory located in Berkeley, California. It conducts unclassified scientific research and is managed by the University of California for the U.S. Department of Energy. »Learn more about computing sciences at Berkeley Lab.